Biology Follows Geology: Micro-Topography Influences Intertidal Community Dominance at Santa Cruz Island, California
- Elise Baugh
- Feb 12, 2024
- 14 min read
Updated: Feb 12, 2024
Authors: Elise Baugh, C. Thomas Carey, Nicholas Spritzer | Department of Ecology and Evolutionary Biology, University of California Santa Cruz, Santa Cruz, California, 95060

Abstract
The micro-topographic details of the substrate in an intertidal zone have a distinct impact on the diversity of life present. Previous studies highlight interactions between the intertidal community and their physical structures and interactions, but there is a lack of understanding concerning how the geological of the substrate significantly shape the potential for various life forms to find a foothold and thrive within this each unique habitat. We decided to evaluate whether the substrate's porosity plays a significant role in determining community dominance and analyzed the. size of individual members of sessile communities. In addition, we also investigated bioengineering target species associated with both macro-dominated and micro-dominated communities and found patterns correlated with substrate porosity, with support from previous studies. The data collected from four intertidal sites highlight a significant relationship between porosity and the dominance of sessile communities in the intertidal zone, with the abundance of macro-communities being higher on the more porous substrates. This study provides an insight into sessile community responses to substrate micro-topography, which can aid future research and modeling of how physical microstructures’ in an environment impact on biological communities and vice versa. This study highlights the importance of incorporating management and monitoring of coastal communities and understanding the interaction between biotic and abiotic elements. With rising sea levels becoming a significant threat to both intertidal and coastal communities, this study emphasizes the benefits that invertebrate communities provide in combating coastal erosion due to climate change.
Key Words: Intertidal, geomorphology, micro topography, porosity, barnacles, mussels, sessile species, Santa Cruz Island, Channel Islands, hydrodynamics, community habitat study, Santa Cruz Island volcanic substrate, bioengineering, costal erosion, invertebrate, intertidal zone
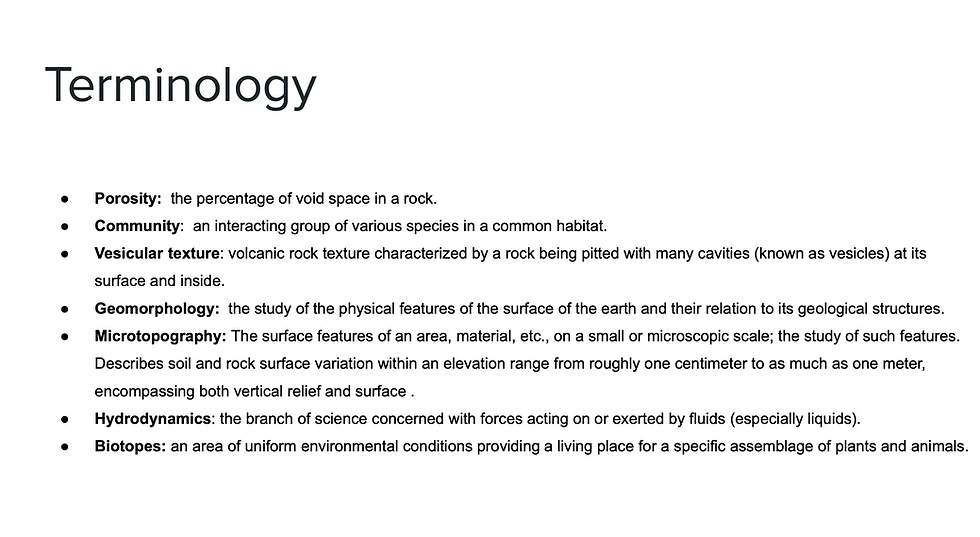
Introduction:
Geomorphology shapes the substrates that then facilitate habitable intertidal communities. Various yet distinct tectonic interactions that shaped Santa Cruz Island provide a unique opportunity to study the influences of geomorphology on community structure (Davis et al. 2020). Therefore, we chose to study the variation in porosity as the geomorphological characteristic of intertidal communities. We compared the size of communities on porous rocks to nonporous rocks at the same study site (figure 7). To optimize our time spent sampling, we categorized species into microorganisms with organisms that are less than half of a centimeter above the substrate and macroorganisms with heights greater than half of a centimeter above the substrate. We hypothesized that more porous substrates would facilitate a higher percent cover of macro-communities than less porous surfaces.
We aligned our research with previous habitat studies that investigated the roles of abiotic and biotic interactions in shaping the structure of communities rather than studies that focused on a specific species or biological interaction (Raman et al. 2013). The relationship between substrate and colonizer is more dynamic than initially anticipated (Coombes et al. 2017). Substrates affect the community of organisms that can colonize an area. These colonists also prevented patterns of erosion on the substrates where settlement occurred. This study emphasized the importance of future research that highlights the dynamic relationship between the substrate and its unique biological community within the intertidal zones, unveiling the invertebrate's unique role in protecting coastal habitats.
Macro-Communities:
The most abundant species observed in our macrocommunities (figure 4) were: Mussel (Mytilus californianus), Aggregating anemone (Anthopleura elegantissima), Solitary green anemone (Anthopleura sola), Giant green anemone (Anthopleura xanthogrammica), barnacles (Tetraclita rubescens), Gooseneck barnacles (Pollicipes polymerus or Balanus glandula), and Seagrasses (Zostera marina)
Micro-Communities:
The most abundant species observed in our micro-communities were acorn barnacles (Balanus glandula) and red algal tuft (Gelidium coulteri). We chose mussels (Mytilus californianus) as a target species of macro communities and acorn barnacles (Balanus glandula) as a target species for micro-communities. Our research goal was to contextualize the relationship between substrates and their bioengineering community members. In addition to their high abundance across sample sites, we chose mussels for our substrate and macro-community structure analysis based on previous studies that found the average length of mussels to be higher on more porous substrates (Brenner and Buck 2010). Acorn barnacles and micro red algae were chosen as target species for microorganisms due to their ability to decrease erosion of the substrates they settled on (Liang et al. 2018; Qureshi et al. 2022).
Invertebrates as bioengineers
The relationship between bioengineering species and substrate types in the intertidal is a valuable but understudied role requiring more research (Baxter et al. 2022). Members of both macro and micro-Organisms utilize adhesive adaptations to succeed in the intertidal. These adhesive strategies can then influence the erosion patterns of their substrate. Geomorphology focuses on large timescales which can limit research. Studying specific bioengineering organisms and their roles in altering substrate erosion is an efficient way to explore the relationship between biology and geology while maintaining realistic time, effort, and expense study parameters.
Research Methods:
Physical Setting of Study Site:
Santa Cruz Island is located 35 km south of Santa Barbara, California (Blanchette, 2006) and is the largest northern California Channel Islands. We selected four intertidal research sites distributed around the island (figure 6). Two surveyed sites, Forney and Fraser Point, are on the western side of Santa Cruz Island, and the remaining two, Willows Cove and Valley Anchorage, are on the eastern side. We chose these sites based on variations in geologic formations, logistical constraints, property access, past research, and representative distribution across the island. SCI is considered a "dynamic oceanographic setting" positioned between two opposing water masses (Blanchette 2006). Santa Cruz Island, along with the other Channel Islands such as San Miguel and Santa Rosa, is highly influenced by the Point Conception upwelling system, which brings colder waters to the westernmost islands. Conversely, the easternmost islands, such as Anacapa Island and the eastern side of Santa Cruz Island, are characterized by warmer waters brought by the Southern California Countercurrent. In addition, geology illustrates the island's natural history with formations of porous volcanic substrates, nonporous sedimentary rocks, and terrestrial deposits across the island.
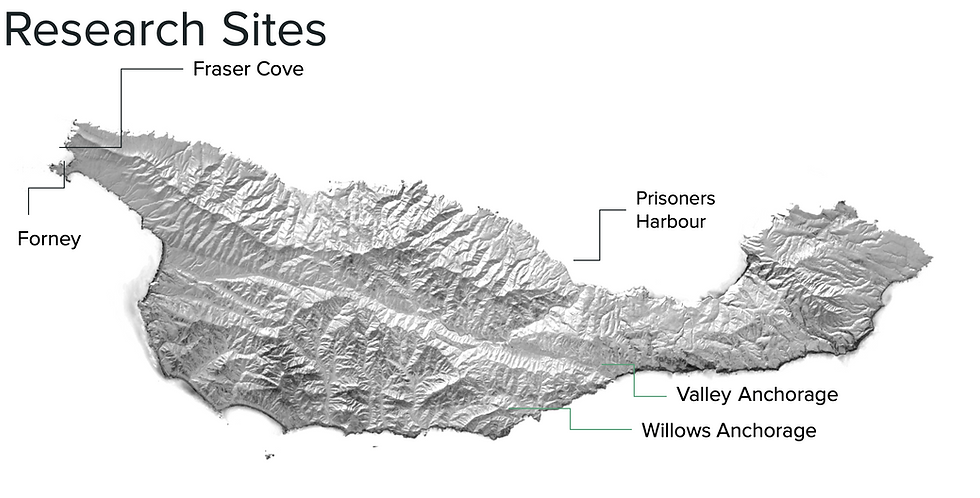
GPS coordinates for the 4 sites around the island:
Fraser, Santa Cruz Island, California, USA | -119.91944 | 34.0625 |
Forney, Santa Cruz Island, California, USA | -119.92222 | 34.0563889 |
Willows, Santa Cruz Island, California, USA | -119.755 | 33.9619444 |
Valley, Santa Cruz Island, California, USA | -119.66583 | 33.9838889 |
Prisoners, Santa Cruz Island, California, USA | -119.68694 | 34.02 |
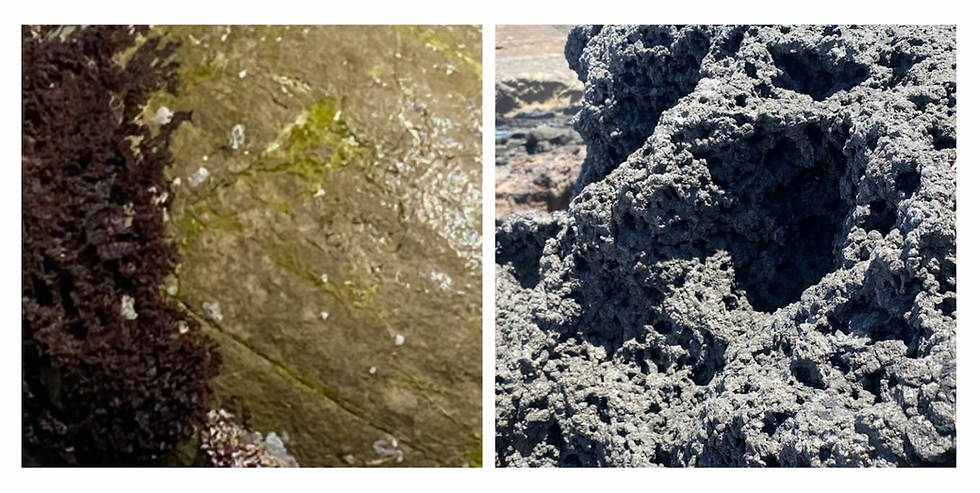
Defining Porosity:
We described porous surfaces as substrates that formed or eroded in a way that left indentations across the majority of the surface (figure 5). Indentations, or vesicles, on porous substrates were unique and varied based on size, topography, and distribution. Substrates with high porosity were often volcanic. Volcanic formations were observed in all sampling sites but were most abundant at Fraser and Forney, the two western research areas. We defined nonporous surfaces as substrates that formed or eroded in a way that left smooth, flat, shelf-like surfaces (figure 5). Rock types that produced smooth flat substrates were primarily sandstone, mudstone, or granitic substrates that lacked observable concavity.
Micro and Macro-Organism Surveys:
We surveyed micro and macro-organisms at four intertidal sites. All sites contained both porous and nonporous substrates. Surveys were conducted using belt transects and point quadrats during the daily low tide at two different tidal zones, specifically the middle and the low tidal line. We determined the low tidal zone as the waterline at low tide. We determined the mid tidal zone as half of the distance between the waterline and the end of the observed biological cover. We measured percent cover by laying a 10 m transect at mid and low tidal zones. Transects were positioned horizontally to the tidal line at each site. We used a 16-point 0.5m x 0.5m quadrat every 1m mark to document instances of macro and microorganisms. We categorized species into the nominal groupings of microorganisms or macroorganisms defined by height. If the species' height above the substrate level was less than 0.5 cm, we categorized the individual as a microorganism. We categorized a species as a macro-organism if the individual's height was greater than 0.5 cm.

Defining Community Dominance:
We distilled the differences between the mean percent cover of each community into nominal categories to further establish a measurement of community dominance based on substrate. Nominal categories included microorganism dominance, macro-organism dominance, co-dominance, or no-dominance (bare substrate.) When characterizing dominance by a microorganism or a macro-organism, the difference in percent cover was greater than 15%.
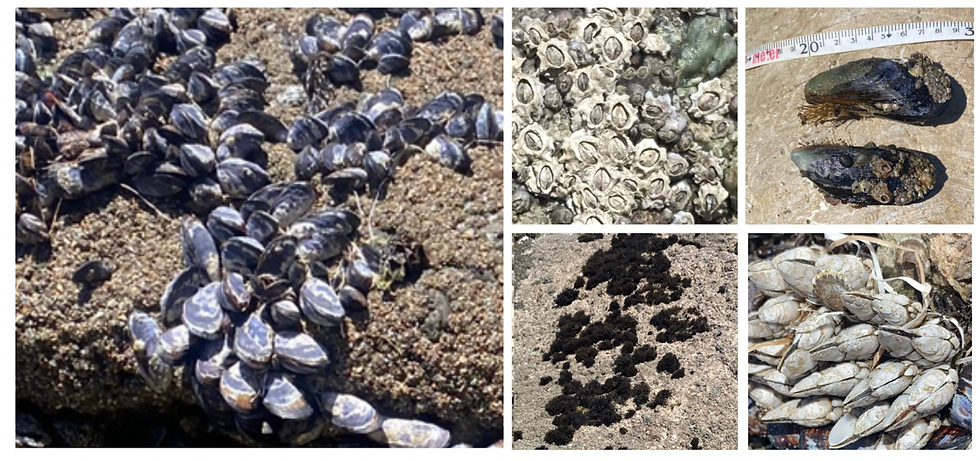
Maximum Mussel Length Sampling:
We surveyed maximum mussel lengths by collecting measurements of the tallest mussels at each transect length to evaluate the impact of the substrate porosity on the size of mussels. We sampled mussel lengths at both mid and low tidal zones using rulers and transect tapes to measure the maximum heights of 3 mussels per substrate type at each site.
Statistical Analyses:
To explore the association between the porosity of the substrate and the size of dominant community members in the intertidal community, we utilized JMP®, Version <x>. SAS Institute Inc., Cary, NC, 1989–2021. Through JMP, we performed two-sample T-tests to compare the mean percent cover of micro and macroorganisms across porous and nonporous substrates. In addition, we created a contingency table based on nominal dominance categories and designed a chi-square that evaluated the macro and micro organism dominance on porous and nonporous surfaces.
Results
Mean Percent Cover:
The mean percent cover of macro-organisms was significantly higher on porous substrates than on nonporous substrates (T2(157.1583, 0.05) = 23.01409, P= <.0001)(figure 1). The data supported our hypothesis that porous substrates would facilitate a higher percent cover of macroorganisms. Additionally, the mean percent cover of microorganisms was higher on less porous substrates (T2(116.5537 ,0.05) = 12.4662, P= <.0001). For further context as to variance in community structure associated with substrate porosity, the mean percent cover of bare space, or no biological cover, was also higher on nonporous substrates compared to porous substrates (T2(98.86062, 0.05) = 6.42753, P= <.0001).

Community Dominance:
A chi-square test showed instances of macroorganism -community dominance was higher on porous substrates, whereas microorganism dominance was higher on nonporous substrates (X2(115, 0.05) = 112.493, P= <.0001)(figure 2).
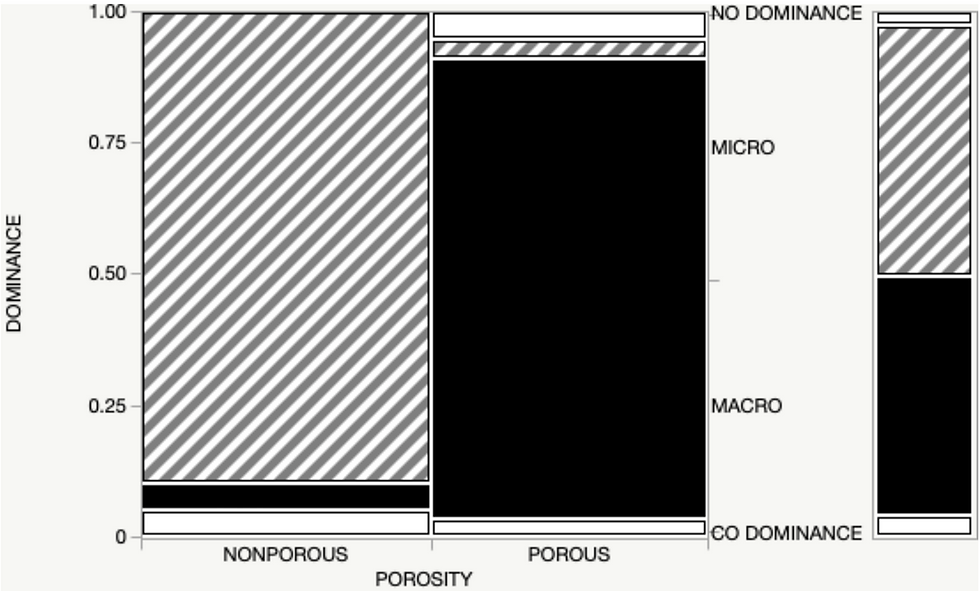
Mussel Length:
We found that the maximum length of mussels was significantly longer on porous substrates than on nonporous substrates (T2(19.47676, 0.05) = 6.926309, P= <.0001) (figure 3).
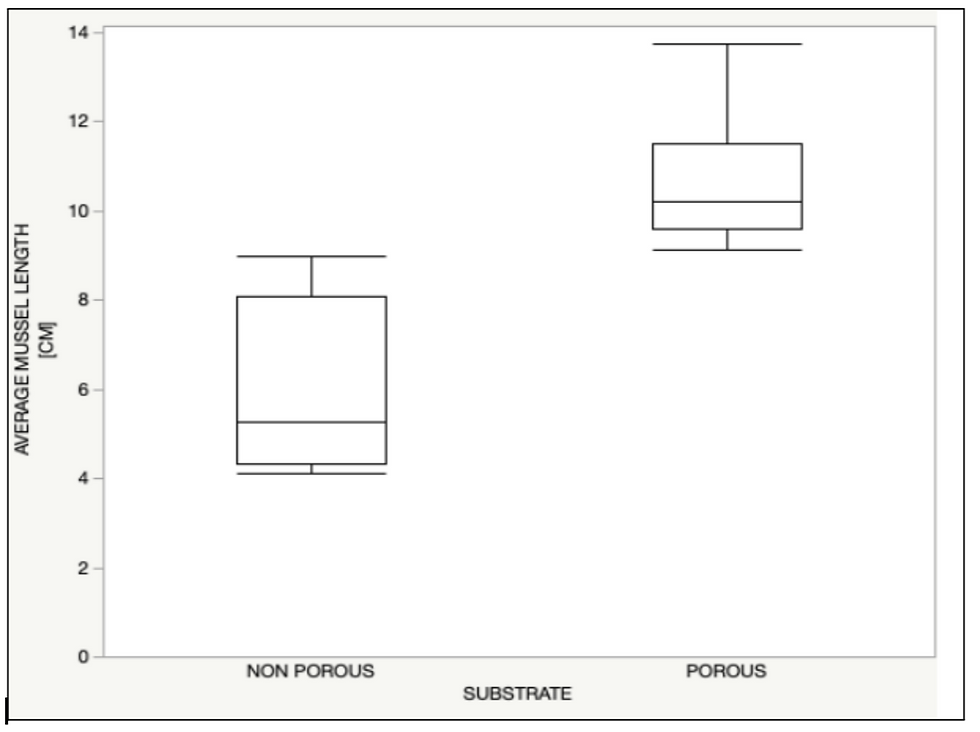
Discussion:
As human development and climate change restructure coastal ecosystems, monitoring the abiotic and biotic dynamics that influence erosion will become increasingly fundamental in developing our coastlines sustainably. We found a correlation between substrate porosity and macroorganism community dominance which is echoed in previous literature (Wallenstein and Neto 2006). We also found results that supported previous studies regarding the bio-engineering capabilities of a target species in our macro organism community (M. californicus) and a target species in our microorganism community (B. glandula) (Coombes et. al 2017). Intertidal habitat studies like ours can contribute insights into the community patterns associated with different substrates and the effect on erosion that these communities cause. These patterns will help inform responsible human development when planning for the impacts of climate change, but they will also help utilize ecosystem services that these biotopes provide. Our work confirmed that modeling erosion based on the dynamics between the substrate and the communities that these substrates facilitate is an efficient way to ensure safe human development and preserve the ecosystem services of intertidal communities.
Micro-Organisms Dominate Nonporous Substrates:
We observed a correlation between nonporous substrates and microorganism dominance. We attribute this relationship to differences in the support and permeability associated with different substrate types. Non-porous substrates offer less structural support to organisms, making nonporous communities more susceptible to high-intensity wave action (Lavallée et al. 2021). Despite the exposure to harsh environmental disturbances, our results demonstrated that microorganisms dominated these substrates successfully (figure 1). The dominance of nonporous substrates by microorganisms, including acorn barnacles and tuft algae, may be explained by the unique adaptations that both species have developed to help grip less porous substrates.
While acorn barnacles appeared to be widely successful, they did seem to have a preferred geometry, chemistry, and rigidness required from the substrate to achieve the necessary adhesion (Petersen et al. 2020). Adhesive properties help barnacle species attach to a wide range of substrate types and maintain a competitive edge in the highly contested struggle for space in the intertidal (Connell 1961). While the genetic mechanisms responsible for barnacle adhesion, or "cement", are beyond the scope of this study, the effect of adhesion on the competitive ability of barnacle species is a significant factor in shaping intertidal zonation and influencing substrate erosion (Liang et al. 2018). Dense aggregating species like barnacles or tuft algae could influence the microclimates of the substrate that the species attaches to, which then decreases the rate of erosion on favorable substrates.
Previous studies also showed that algal species serve as pioneer species in rocky intertidal zones by colonizing less habitable substrates. These early successional organisms also benefit future communities by altering microclimates. The presence of these species engineers ecosystems further by modifying erosion rates and dissipating wave energy (Denny 2021). When they die, they become detritus and form the basis of the food chain serving as a food source for filter feeders such as barnacles (Greene et al. 2016). Recent studies even demonstrated that community structures and diversity could often be dependent on a particular dominant algal species (Lily and Schiel 2005). While microorganisms dominate nonporous substrates, we saw a lack of microorganism dominance on porous surfaces (figure 2). These results suggested that this substrate fails to meet ideal conditions for microorganisms to adhere to or recruit. (Buasakaew et al. 2021) discovered similar results and attributed the lack of dominance to water temperature and salinity, two areas with potential links to the substrate porosity.
Macro-Organisms Dominate Porous Substrates:
Macro-organism communities dominated porous substrates and appeared to benefit from the physical support of Santa Cruz Island volcanic substrates. Mussels grip the substrates using "feet'' called byssi, which produce adhesives and facilitate mussels' zonation in high-intensity wave action intertidal areas (Qureshi et al. 2022). Brenner and Buck explained that the pattern of increasing the length of mussel shells across more porous substrates results from a mussel's ability to grip a substrate. A mussel may attach itself to a substrate initially; however, if a mussel grows too big, the substrate will not provide sufficient grip to protect the increasingly large mussel from wave action, and the individual will be dislodged and carried out to sea. This research corresponds with our data analysis indicating substrates with higher porosity will facilitate mussels of longer shell lengths (figure 3). Our study also underscored that hydrodynamic-driven sediment disturbances challenge macroorganisms in early and late-stage life histories. Our analysis suggested that porous structures may play a role in protecting macroorganism communities from wave action and sediment disturbances through the inherent structural support of the substrate. Dependence on porous substrates for structural protection would also explain why we saw nonporous substrates dominated by less dependent micro-communities.
Implications:
The dynamics between biotic and abiotic components of the intertidal zone can influence each other (Denny 2021). The advancement of tools to measure abiotic components of the intertidal will make it possible to conduct more inclusive habitat studies focused on the effect of abiotic components that were previously difficult to measure consistently. Substrate porosity and wave action are analyzed with greater precision due to technical advancements in data modeling. Modeling erosion based on the substrate's relationships with biotic communities will enable accurate predictions. These communities preserve substrates by removing moisture, salt, and other deteriorative agents and modifying microclimates (Liang et. al 2018). In the face of climate change, bioengineering capabilities of intertidal communities have significant potential for the future of green infrastructure.
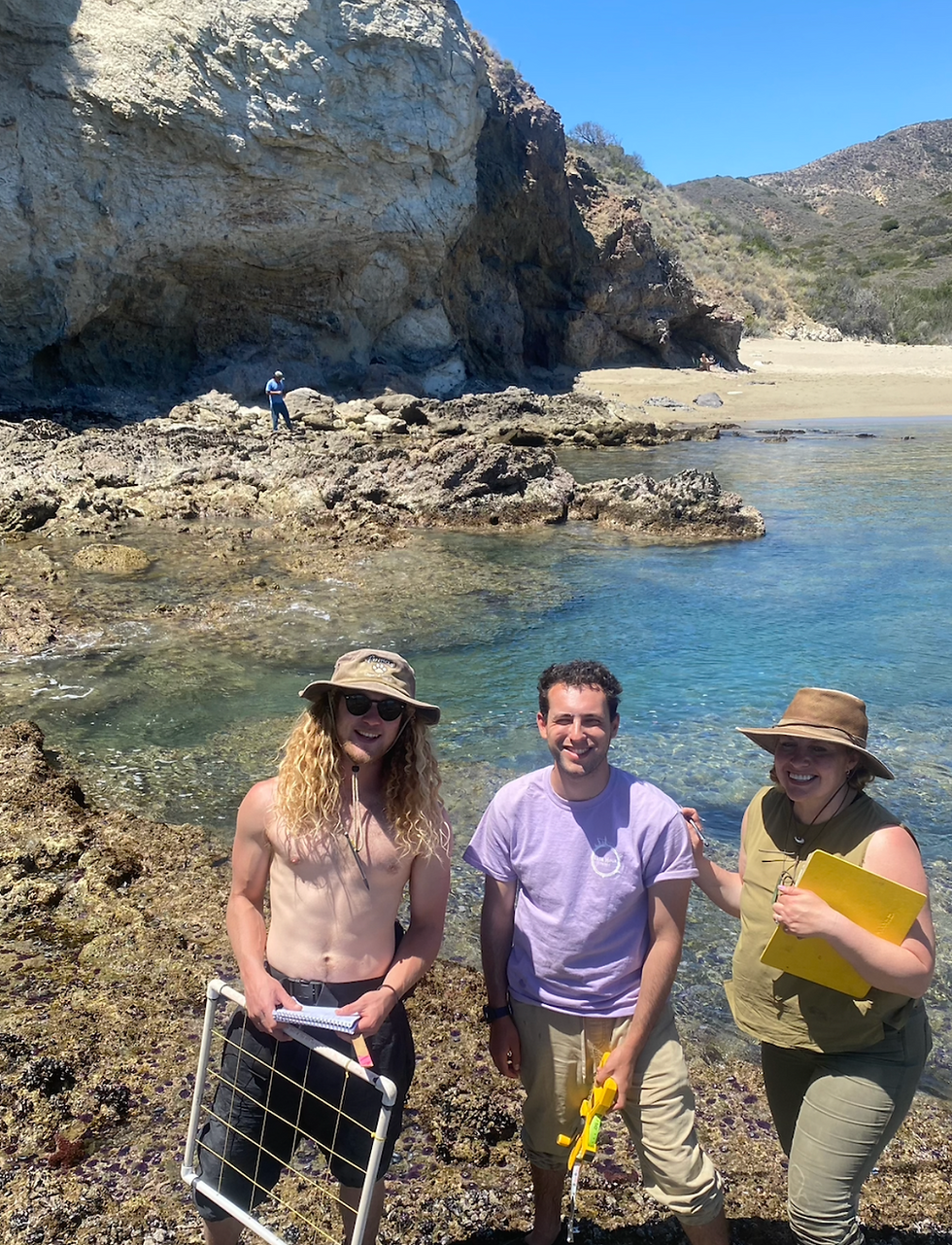
Conclusion:
Climate change scientists project an additional 22-25 cm of sea-level rise in the next 30 years. Predictions include an upward trend in high-intensity storm surges and a greater frequency of extreme weather events. These changes present a clear danger that continues to threaten our coastal regions (Barbier 2020). Understanding the roles that macro and micro organism marine communities serve in protecting the structural integrity of our coastline will serve as a tool against coastal erosion and habitat destruction (Denny 2021). These biotopes have the potential to reduce wave energy intensity, prevent flooding damage (Barbier 2020), and serve as a natural green infrastructure (Koch et al. 2009). Incorporating intertidal communities into green infrastructure to reduce erosion naturally has the potential to sustain the bio-engineering services of these communities and save developers money over time.
Experimental Challenges and Future Recommendations:
Challenges and errors in our methods included probable miscounts of micro or macro-organisms, and dealing with multiple layers of organisms may have led to the misclassification of substrate types. We also dealt with the logistics of island travel and approximated times at which low tide will be prominent, and the shortening of the amount of time needed to conduct sampling on the island should be considered when conducting future research at intertidal sites. Extensive sampling should occur seasonally to understand cyclic changes of intertidal organisms. In addition, since dynamics between biotic and abiotic components of the rocky intertidal can influence each other, this analysis has brought to light the need for further study. This research demonstrates that the rocky intertidal substrates are serving as habitats that protect from wave energy and harbor biota from predation, and prevent sediment disturbance while maintaining permeability. This study requires further steps to gain a deeper understanding of how the biota impacts the substrate. Studies have demonstrated that these communities serve as bio protectors of the substrates (Liang et al. 2018). They preserve the structural integrity by removing moisture, salt, and other deteriorative agents and modify microclimates. The advancement of tools to measure abiotic components of the intertidal will make it possible to conduct more inclusive habitat studies focused on the effect of abiotic components that were previously impossible to measure consistently.
Climate change scientists project an additional 22-25 cm of sea-level rise in the next 30 years. Predictions include an upward trend in high-intensity storm surges and a greater frequency of extreme weather events. These changes present a clear danger that continues to threaten our coastal regions (Barbier 2020). Understanding the roles that macro and micro organism marine communities serve in protecting the structural integrity of our coastline will serve as a tool against coastal erosion and habitat destruction (Denny 2021). These biotopes have the potential to reduce wave energy intensity, prevent flooding damage (Barbier 2020), and serve as a natural green infrastructure (Koch et al. 2009). Incorporating intertidal communities into green infrastructure to reduce erosion naturally has the potential to sustain the bio-engineering services of these invertebrate communities and save developers money over time.
Acknowledgments:
We appreciate our access to the reserve and thus our ability to conduct research sampling, and would like to acknowledge the UC Natural Reserve System, the Nature Conservancy, and its stewards. We also thank Professors Don Croll and Gage Dayton, Rachel Pausch, Kaila Pearson, and Jenna Sparks, for their assistance and guidance throughout our time at Santa Cruz Island. Finally, we would like to thank Dr. Carol Blanchette and Dr. Lyndal Laughrin for sharing their Santa Cruz Island wisdom.
Literature cited:
Barbier, Edward B. ``Estuarine and Coastal Ecosystems as Defense Against Flood Damages: An Economic Perspective.” Frontiers in Climate 2 (December 18, 2020): 594254.consistently.
Baxter, T. I., M. A. Coombes, and H. A. Viles. 2022. The bioprotective properties of the Blue Mussel (mytilus edulis) on intertidal rocky shore platforms. Marine Geology 445:106734.
Blanchette, C.A., Broitman, B.R. & Gaines, S.D. Intertidal community structure and oceanographic patterns around Santa Cruz Island, CA, USA. Mar Biol 149, 689–701 (2006).
Brenner, M., and B. H. Buck. 2010. Attachment properties of blue mussel (mytilus edulis L.) byssus threads on culture-based artificial collector substrates. Aquacultural Engineering 42:128–139.
Buasakaew, Nutcha, Benny K. K. Chan, and Kringpaka Wangkulangkul. “Why Are Barnacles Common on Intertidal Rocks but Rare in Rock Pools? Effect of Water Temperature, Salinity, and Continuous Submergence on Barnacle Survival in Indian Ocean Rock Pools.” Frontiers in Marine Science 8 (September 23, 2021): 688894. https://doi.org/10.3389/fmars.2021.688894.
Coombes, M. A., H. A. Viles, L. A. Naylor, and E. C. La Marca. 2017. Cool barnacles: Do common biogenic structures enhance or retard rates of deterioration of intertidal rocks and concrete? Science of The Total Environment 580:1034–1045.
Davis, Thomas L., Richard J. Behl†, Katie M. O’Sullivan†, Sarah Raskin, and Stephen Bryne. “Santa Cruz Island Field Trip: Geology, History, and Research Opportunities.” In From the Islands to the Mountains: A 2020 View of Geologic Excursions in Southern California, by Richard V. Heermance and Joshua J. Schwartz, 115–63. Geological Society of America, 2020. https://doi.org/10.1130/2020.0059(04).
Denny, M. 2021. Wave-energy dissipation: Seaweeds and marine plants are ecosystem engineers. Fluids 6:151.
Connell, Joseph H. “The Influence of Interspecific Competition and Other Factors on the Distribution of the Barnacle Chthamalus Stellatus.” Ecology 42, no. 4 (October 1961): 710–23. https://doi.org/10.2307/1933500.
JMP®, Version <x>. SAS Institute Inc., Cary, NC, 1989–2021
Lavallée, Yan, and Jackie E. Kendrick. “A Review of the Physical and Mechanical Properties of Volcanic Rocks and Magmas in the Brittle and Ductile Regimes.” In Forecasting and Planning for Volcanic Hazards, Risks, and Disasters, 153–238. Elsevier, 2021. https://doi.org/10.1016/B978-0-12-818082-2.00005-6.
Liang, C., Z. Ye, B. Xue, L. Zeng, W. Wu, C. Zhong, Y. Cao, B. Hu, and P. B. Messersmith. 2018. Self-assembled nanofibers for strong underwater adhesion: The trick of barnacles. ACS Applied Materials & Interfaces 10:25017–25025.
Lilley, S. A., & Schiel, D. R. (2006). Community Effects following the Deletion of a Habitat-Forming Alga from Rocky Marine Shores. Oceologia, 148(4), 672–681. http://www.jstor.org/stable/20445956.
Petersen, Dennis S., Stanislav N. Gorb, and Lars Heepe. “The Influence of Material and Roughness on the Settlement and the Adhesive Strength of the Barnacle Balanus Improvisus in the Baltic Sea.” Frontiers in Marine Science 7 (August 18, 2020): 664. https://doi.org/10.3389/fmars.2020.00664.
Qureshi, D. A., S. Goffredo, Y. Kim, Y. Han, M. Guo, S. Ryu, and Z. Qin. 2022. Why mussel byssal plaques are tiny yet strong in attachment. Matter 5:710–724.
Raman, S., L. Karunamoorthy, M. Doble, R. Kumar, and R. Venkatesan. 2013. Barnacle adhesion on natural and synthetic substrates: Adhesive structure and composition. International Journal of Adhesion and Adhesives 41:140–143.
Ruckelshaus, Mary H., Gregory Guannel, Katherine Arkema, Gregory Verutes, Robert Griffin, Anne Guerry, Jess Silver, Joe Faries, Jorge Brenner, and Amy Rosenthal. “Evaluating the Benefits of Green Infrastructure for Coastal Areas: Location, Location, Location.” Coastal Management 44, no. 5 (September 2, 2016): 504–16. https://doi.org/10.1080/08920753.2016.1208882.
Comentários